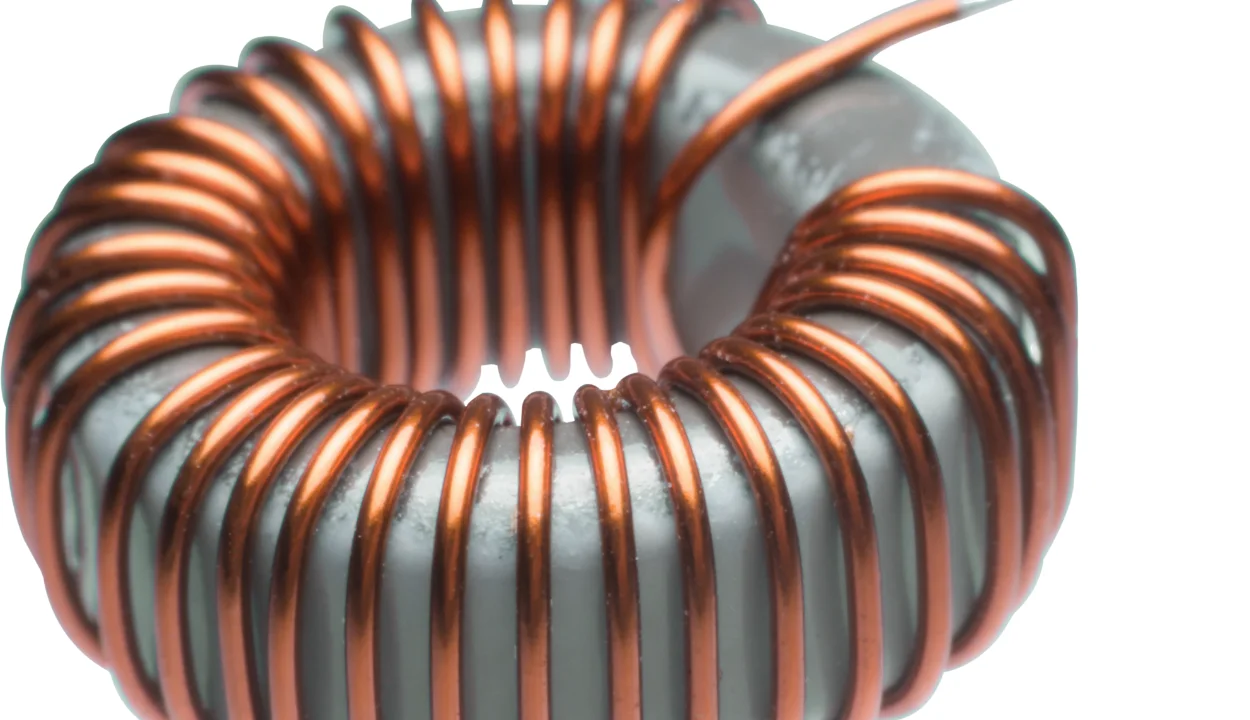
Introduction
Inductors are fundamental components in the world of electronics. They’re at the heart of countless applications, from power supply circuits to radio transmitters. But what exactly is an inductor, and how does it transform electrical energy into magnetic energy—only to return that energy back to the circuit when needed?
Whether you’re a seasoned electrical engineer, an electronics hobbyist, or simply curious about the science behind inductors, this guide will break everything down for you. By the end, you’ll understand the science, applications, and nuances of inductors like never before.
What is an inductor?
An inductor, at its core, is a passive electronic component that stores energy in a magnetic field when electric current flows through it. Essentially, it’s a coil of wire, and its inherent ability to resist changes in current is what makes it unique.
Inductors are defined by their inductance, measured in henries (H). Inductance is a property that quantifies how effectively an inductor can convert electrical current into a magnetic field.
Key Components and Characteristics:
-
Coil of Wire: Wound in a spiral, this is the foundation of an inductor.
-
Core Material: This could be air, ferrite, or other materials to enhance magnetic properties.
-
Inductance (L): The measure of an inductor’s ability to store energy.
-
Resistance (although small) can influence energy loss.
Typical inductors might look simple, but the underlying physics is fascinating. Let’s dive deeper into how they work.
How does an inductor work?
Inductors operate under Faraday’s Law of Electromagnetic Induction. Whenever current flows through a conductor (the wire coil in this case), a magnetic field forms around it.
When the current changes, the magnetic field evolves, inducing a voltage (or electromotive force) that opposes the change in current. This is referred to as self-inductance, a feature that makes inductors particularly useful for controlling current in a circuit.
Dual Energy Exchange:
-
Energy Storage:
When current flows, an inductor stores energy in its surrounding magnetic field.
Formula for energy stored:
E = ½ L I²
(where L is inductance and I is current).
-
Energy Release:
When the current decreases, this magnetic field collapses, converting back into electrical energy and delivering it to the circuit.
Why do inductors oppose changes in current?
Inductors resist changes in current flow due to the opposition invoked by their electromagnetic properties. When the current increases, the inductor “pushes back” with an opposing voltage. Similarly, when current decreases, the inductor delivers its stored energy to maintain flow. This property is critical for smoothing current variations in power supplies.
Key Parameters That Define Inductor Performance
Not all inductors are created equal! Their behavior in different circuits depends on several parameters, including the following:
1. Inductance (L):
The ability of the inductor to store energy and resist changes in current is important. The larger the inductance, the greater its resistance to current fluctuations.
2. Core Material:
The material inside the wire coil—typically ferrite, iron, or air—affects the inductor’s ability to concentrate the magnetic field. For instance, ferrite cores boost efficiency in high-frequency circuits.
3. DC Resistance (DCR):
Though inductors are mainly designed for storage, they may exhibit small resistive qualities, leading to energy dissipation as heat.
4. Saturation Current:
Inductors have a maximum current threshold. Exceeding this can cause the magnetic field to saturate and reduce performance.
Understanding these specifications helps engineers custom-tailor inductors for various applications.
Applications of Inductors in Electronics
1. Power Supplies:
Inductors smooth voltage and reduce ripple in power systems, typically working alongside capacitors to form filter networks.
2. Transformers:
Inductors in transformers step voltages up or down for electrical grids and appliances.
3. RF Circuits:
Radio frequency circuits use inductors to tune signals, helping to select specific frequencies for transmission or reception.
4. Energy Storage:
Inductors store energy temporarily in converters, such as inductor-based DC-DC voltage regulators.
5. Chokes:
Used to block high-frequency AC signals, preventing interference without affecting DC.
From your smartphone to electric vehicles, inductors play a quiet yet pivotal role in modern advancements.
Types of Inductors and When to Use Them
Inductors come in a variety of shapes and sizes tailored to specific needs. Here are the most common types:
1. Air-Core Inductors:
Best for low-frequency applications or where minimal inductance is required, as it lacks a magnetic core material.
Use case: Tuned circuits, like radios.
2. Iron-Core Inductors:
With an iron core, these inductors offer higher inductance compared to air-core versions.
Use case: Power supplies and audio applications.
3. Ferrite-Core Inductors:
Ferrite materials allow inductors to perform exceptionally well at high frequencies. They’re efficient and compact.
Use case: RF and high-frequency switching circuits.
4. Toroidal Inductors:
These inductors have donut-shaped cores, which minimize energy losses and leakage thanks to their magnetic field containment.
Use case: High-efficiency power supplies.
5. Multilayer Chip Inductors:
Compact and surface-mountable, they are widely used in PCB designs for compact devices like smartphones.
Use case: mobile technology and space-constrained circuits.
Each type of inductor fulfills a unique purpose, making it essential for engineers to select the right one based on the application and frequency range.
Challenges and Limitations of Inductors
While inductors are indispensable, they’re not without their challenges:
-
Bulky Size for High Values:
High-inductance inductors often require large, cumbersome coils.
-
Losses Due to Resistance:
The DCR of a wire coil can lead to unwanted energy loss as heat.
-
Magnetic Interference:
Proximity to other components within a circuit can lead to magnetic coupling issues.
Efforts continue to optimize inductor design for modern needs, such as developing nanomagnetic or graphene-based inductors. The future of inductors looks promising.
Advancing Efficiency with Inductors
With the rise of IoT, renewable energy, and miniaturized electronics, the efficient design and application of inductors are more critical than ever. Engineers are exploring new core materials, enhanced winding techniques, and hybrid inductor technologies to meet growing demands for performance and compactness.
For instance, modern applications like inductive wireless charging and high-efficiency LED lighting heavily rely on advancements in inductor technology.
Transform Your Designs with Inductors
Inductors unlock endless possibilities for managing electrical energy in advanced systems. From tuning radio frequencies to stabilizing power supplies, their ubiquitous presence often goes unnoticed yet remains irreplaceable.
Understanding how inductors function, their parameters, and practical applications empowers you to design better circuits and solve real-world challenges efficiently.
Whether you’re fine-tuning a power supply or building the next great innovation, mastering inductors is a critical step toward creating a robust and scalable solution.